RESEARCH - Henning Mootz
Protein chemistry / biotechnology
Coordination in multi-domain biosynthesis enzymes
The biosynthesis of complex natural products is one of the most fascinating aspects of protein function from a chemists' perspective. Multi-step reactions are orchestrated by multiple enzymes that catalyze the synthesis from simple precursors. Arguably, the producing microorganisms or plants can be regarded as excellent synthetic chemists. We are interested in the biosynthesis of a certain class of natural products, the nonribosomal peptides. This group of natural products include important compounds like the penicillins, vancomycin and cyclosporine (Figure 1).

They can display antibiotic, antifungal, and immunosuppressive activites, for example. According to their name, these are not synthesized in an mRNA-templated fashion on the ribosome, but instead, on protein templates called nonribosomal peptide synthetases (NRPS). Nonribosomal peptides are not limited to the 20 proteinogenic amino acids but can contain a huge number of different building blocks as well as various modifications such as lipidation or glycosylation. Furthermore, most peptides are cyclic or branched cyclic to increase their bioactivity. NRPSs synthesize their products in a manner reminiscent of an assembly-line (Figure 2).
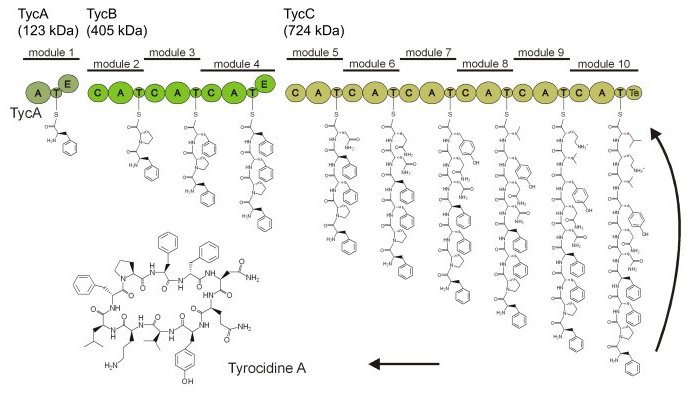
NRPSs are composed of modules, each of which is typically responsible for the incorporation of one amino acid into the growing peptide chain (1). Modules can be further subdivided into single domains that harbour the catalytic activities, for example the adenylation domain selects an amino acid and activates it to the aminoacyl adenylate under consumption of ATP, or serve as carrier domains to covalently bind the intermediate as a 4'-phosphopantetheinyl thioester.
We are interested in how these mega-enzymes work. How is a directed coordination between the multiple domains achieved? For example, the NRPS responsible for the biosynthesis of the cyclic decapeptide antibiotic tyrocidine consists of 32 domains, which are located on three subunits TycA, TycB, and TycC (Figure 2)(Mootz & Marahiel, 1997). Although crystal structures of isolated domains of each type and even of entire modules are known, their interplay in space and time is not well understood. Each peptidyl-carrier protein (PCP) or thiolation domain has to interact with several catalytic centers, however, these are too far apart to be reached by a simple movement of the 4'phosphopantetheine prosthetic group. Consequently, significant conformational changes and domain movements are postulated.
To address these questions, we use a combination of biochemical, chemical biology and biophysical techniques. We study conformational dynamics of purified and reconstituted multi-domain ensembles in solution and in real time under conditions of catalysis, for example by monitoring intramolecular distance changes between domains using FRET (Alfermann et al., 1997). We aim to decipher the importance and contribution of conformational changes (conformational control) and how this is coupled to catalysis mediated by the specificity of the domains for the different intermediates bound at the A domain and presented on the PCP (catalytic control). Using substrates, ligands and proteins engineering with photoactivatable amino acids (Rüschenbaum et al., 2022) we selectively perturb the investigated model NRPS systems, simulate defined intermediary states and trigger reactions. We delineate the individual stages of the multi-step synthesis and piece them together to the stepwise conformational shifts associated with the multi-domain interplay in the overall biosynthesis (Figure 3).

Selected publications
Feldberg, A.-L., Mayerthaler, F., Rüschenbaum, J., Kröger, J., Mootz, H. D.
Carrier Protein Interaction with Competing Adenylation and Epimerization Domains in a Nonribosomal Peptide Synthetase Analyzed by FRET.
Angew. Chem. Int. Ed., 2024, published online, doi: 10.1002/anie.202317753.
Selected as a “hot” paper
Sun, X., Alfermann, J.*, Li, H.*, Watkins, M. B., Chen, Y.-T., Morrell, T. E., Mayerthaler, F., Wang, C.-Y., Komatsuzaki, T., Chu, J.-W., Ando, N., Mootz, H. D.,* Yang, H.*
Sub-Domain Dynamics Enable Chemical Chain Reactions in Nonribosomal Peptide Synthetases.
Nat. Chem., 2024, 16, 259-268, doi: 10.1038/s41557-023-01361-4.
Rüschenbaum, J., Steinchen, W., Mayerthaler, F., Feldberg, A.-L., Mootz, H. D.
FRET Monitoring of a Nonribosomal Peptide Synthetase Elongation Module Reveals Carrier Protein Shuttling Between Catalytic Domains.
Angew. Chem. Int. Ed., 2022, 61, e202212994. doi.org/10.1002/anie.202212994.
Mayerthaler, F., Feldberg, A.-L., Alfermann, J., Sun, X., Steinchen, W., Yang, H., Mootz, H. D.
Intermediary conformations linked to the directionality of the aminoacylation pathway of nonribosomal peptide synthetases.
RSC Chem. Biol., 2021, 2, 843-854, doi: 10.1039/d0cb00220h.
Dehling, E.,* Rüschenbaum, J.,* Diecker, J., Dörner, W., Mootz, H. D.
Photo-crosslink analysis in nonribosomal peptide synthetases reveals aberrant gel migration of branched crosslink isomers and spatial proximity between non-neighboring domains.
Chem. Sci., 2020, 11, 8945-8954, doi: 10.1039/D0SC01969K.
Reille-Seroussi, M., Mayer, S. V., Dörner, W., Lang, K., Mootz, H. D.
Expanding the genetic code with a lysine derivate bearing an enzymatically removable phenylacetyl group.
Chem. Comm. (Camb), 2019, 55, 4793-4796, doi: 10.1039/c9cc00475k.
Alfermann, J., Sun, X., Mayerthaler, F., Morrell, T. E., Dehling, E., Volkmann, G., Komatsuzaki, T., Yang, H., Mootz, H. D.
FRET monitoring of a nonribosomal peptide synthetase.
Nat. Chem. Biol., 2017, 13, 1009-1015. DOI:10.1038/nchembio.2435.
Dehling*, E., Volkmann*, G., Matern, J. C. J., Dörner, W., Alfermann, J., Diecker, J., Mootz, H. D.
Mapping of the communication-mediating interface in nonribosomal peptide synthetases using a genetically encoded photocrosslinker supports an upside-down helix-hand motif.
J. Mol. Biol., 2016, 428, 4345-4360. DOI:10.1016/j.jmb.2016.09.007.
Zettler, J., Mootz, H. D.
Conformational changes in the cross-talk between adenylation and peptidyl-carrier protein domains of nonribosomal peptide synthetases.
FEBS J. 2010, 277, 1159-1171.
Mootz, H. D., Marahiel, M. A.
The tyrocidine biosynthesis operon of Bacillus brevis: Complete nucleotide sequence and biochemical characterization of functional internal adenylation domains.
J. Bacteriol. 1997, 179, 6843-6850
Last updated 04/2024