Molecular basis of neuronal differentiation
The establishment of a polarized morphology and functionally distinct axonal and dendritic compartments are essential steps in the differentiation of neurons. During the development of the mammalian neocortex, neural progenitors located in the ventricular zone (VZ) generate successive waves of neurons that form its characteristic six-layered structure. The radial glia cells (RGCs) serve as both neuronal progenitors and substrate for neuronal migration (Figure 1). Newly generated neurons first assume a multipolar morphology in the subventricular (SVZ) and lower intermediate zone (IZ). After several hours they become bipolar in the upper IZ by extending an axon and forming a leading process (Figure 2). This transition from multipolar cells with several dynamic processes to a bipolar neuron is called the multi-to-bipolar (MTB) transition and precedes the radial migration of bipolar neurons along the RGCs into the cortical plate (CP). Defects in this polarization or in neuronal migration are a major cause of neurodevelopmental disorders.
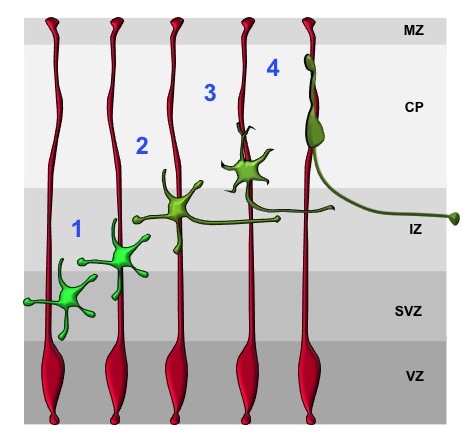
Radial glia cells (RGCs, red) are highly polarized cells that span the embryonic cortex. At the VZ, RGCs are linked by cadherin-dependent adherens junctions and their basal endfeet are attached to the ECM by integrins at the pial surface. Postmitotic neurons (green) transiently assume a multipolar morphology (1). The extension of a trailing (2) and a leading process (3) marks the establishment of polarity. Subsequently, neurons start their radial migration along radial glia processes (red) with a bipolar morphology (4).
To elucidate the signaling pathways that regulate the MTB transition and radial migration of neurons we have established a slice culture system that allows the live cell imaging of migrating neurons after ex vivo electroporation. A central pathway that directs the MTB transition acts through the small GTPases Rap1A and Rap1B. Our analysis of conditional Rap1a;Rap1b;Emx1-Cre double knockout mice shows that Rap1 GTPases are essential for cortical development in vivo. Rap1A and Rap1B act redundantly to maintain the polarity of RGCs and direct the MTB transition in multipolar neurons. The conditional knockout of Rap1 results in a disorganization of RGCs as well as a complete disruption of cortical layers (Figure 3) and a severe reduction of axon formation.

Mouse brains were transfected with an expression vector for membrane-bound EGFP to label early post-mitotic neurons. Imaging of cortical slices shows that multipolar neurons first form an axon and subsequently a leading process.
Live cell imaging of slice cultures after deletion of Rap1a and Rap1b shows that they are required cell-autonomously for the MTB transition. Their loss blocks axon formation and the initiation of neuronal migration and the majority of neurons remain multipolar. We also identified the Rap1 guanine nucleotide exchange factor (GEF) C3G (encoded by the Rapgef1 gene) as a regulator of the MTB transition by conditionally inactivating the Rapgef1 gene in the developing mouse cortex.

The cortex-specific conditional Rap1a;Rap1b;Emx1-Cre knockout shows defects in the polarity of RGCs and neurons. Staining for Tbr2 (marker for intermediate progenitors) reveals defects in the formation of cortical layers. Nestin staining indicates a disorganization of radial glia.
Currently, we are investigating the pathways downstream of Rap1 that mediate its function in the polarization and migration of neurons with a focus on the regulation of intracellular trafficking and membrane dynamics.
Future projects will address the following questions:
How does Rap1 direct the polarization of neurons in vivo?
This question will be investigated using conditional knockout mice, ex vivo electroporation of embryonic mouse brain combined with live cell imaging and ex vivo electroporation.
How do neurons establish polarized transport during neuronal polarization and migration?
The in vivo regulation of membrane dynamics and of polarized transport will be analyzed using conditional knockout mice and live cell imaging of slice cultures
These projects are embedded in the Cluster of Excellence "Cells in Motion" and its graduate program
http://www.uni-muenster.de/Cells-in-Motion/
http://www.uni-muenster.de/Cells-in-Motion/people/all/pueschel-a.php
https://www.uni-muenster.de/CiM-IMPRS/
Selected publications
Ravindran, P., Püschel, A.W. (2022). An isoform-specific function of Cdc42 in regulating mammalian Exo70 during axon formation. Life Sci Alliance. 6, e202201722. doi: 10.26508/lsa.202201722.
Di Meo, D., Ravindran, P., Sadhanasatish, T., Dhumale, P., Püschel, A.W. (2021). The balance of mitochondrial fission and fusion in cortical axons depends on the kinases SadA and SadB. Cell Rep. 37, 110141. doi: 10.1016/j.celrep.2021.110141.
Humpert, I., Di Meo, D., Püschel, A.W., Pietschmann, J.-F. (2021). On the role of vesicle transport in neurite growth: modelling and experiments. Math. Biosci., 338, 108632. doi: 10.1016/j.mbs.2021.108632.
Jin, J., Ravindran, P., Di Meo, D., Püschel, A.W. (2019). Igf1R/InsR function is required for axon extension and corpus callosum formation. PLoS One 14, e0219362. doi: 10.1371/journal.pone.0219362.
Wang, N., Dhumale, P., Chiang, J., Püschel, A.W. (2018). The Sema3A receptor Plexin-A1 suppresses supernumerary axons through Rap1 GTPases. Sci. Rep. 8, 15647.
López Tobón, A., Suresh, M., Jin, J., Vitriolo, A., Pietralla, T., Tedford, K., Bossenz, M., Mahnken, K., Kiefer, F., Testa, G, Fischer, K.-D., Püschel, A.W. (2018). The guanine nucleotide exchange factor Arhgef7/βPix promotes axon formation upstream of TC10. Sci. Rep. 8, 8811.
Dhumale P., Menon S., Chiang J., and Püschel A.W. (2018). The loss of the kinases SadA and SadB results in early neuronal apoptosis and a reduced number of progenitors. PLoS One 13, e0196698.
Shah, B., Lutter, D., Bochenek, M.L., Kato, K. Tsytsyura, Y., Glyvuk, N., Sakakibara, A., Klingauf, J., Adams, R.H., and Püschel, A.W. (2016). C3G/Rapgef1 is Required in Multipolar Neurons for the Transition to a Bipolar Morphology during Cortical Development. PLoS One 11, e0154174.
Shah, B., and Püschel, A.W. (2016). Regulation of Rap GTPases in mammalian neurons. Biol. Chem. 397, 1055-1069.
Shah, B., Lutter, D., Tsytsyura, Y., Glyvuk, N., Sakakibara, A., Klingauf, J., and Püschel, A.W. (2017). Rap1 GTPases Are Master Regulators of Neural Cell Polarity in the Developing Neocortex. Cereb. Cortex 27, 1253–1269.
Dogliotti, G., Kullmann, , Dhumale, P., Thiele, C., Panichkina, O., Mendl, G., Houben, R., Haferkamp, S., Püschel, A.W., and Krahn, M.P. (2017). Membrane-binding and activation of LKB1 by phosphatidic acid is essential for development and tumor suppression. Nat. Comms. 8, 15747.
Shah, B., and Püschel, A.W. (2014). In vivo functions of small GTPases in neocortical development. Biol. Chem. 395, 465-476.